GMP-compliant equipment design: The GMP Equipment Design Guide
What does "GMP-compliant equipment design" mean? This question comes up very often. Mostly, it is companies that have not yet supplied equipment or facility parts to the pharmaceutical industry that ask this question. But the question is also heard again and again at seminars, in this case from representatives of the pharmaceutical companies themselves. And the issue is not limited to the pharmaceutical industry alone. Transformed industries, such as medical device, cosmetics or diagnostics manufacturing, also want GMP-compliant equipment from their suppliers. Inquiries to this effect often cause equipment manufacturers to shrug their shoulders, because the requirements are overloaded, imprecise or simply not correct. But why is that? After all, authorities play an important role in the pharmaceutical industry, from clinical studies to the approval of a drug to GMP inspections. Why is there no regulatory authorization of GMP equipment? Why not a badge comparable to the TÜV?
This is relatively easy to answer. There is no one equipment design that can be compliant for pharmaceutical manufacturing facilities. There are numerous pharmaceutical manufacturing processes, from dry granulation and tableting to fermentation or sterile filling. If you add manufacturing processes from chemical active ingredient production and the production of medical devices (from walking sticks to artificial heart valves), there are many more. And the product itself can also place a wide variety of demands on the production equipment. For example, it makes a big difference to the design of a facility whether the product to be manufactured must be sterile or non-sterile, or whether it is a high-potency product. However, a product or an intermediate product may also be sensitive to oxygen or moisture. This, of course, also affects the design of the equipment. On rare occasions, however, requirements of authorities in different countries may differ. For example, until recently, the method of manufacturing WFI (water for injection) was more heavily regulated in Europe than in the U.S. or Japan. In Europe, production was limited to distillation. A plant producing WFI by reverse osmosis, for example, would thus not have been GMPcompliant in Europe. In other countries, it is still not allowed today. The design of a plant therefore depends, among other things, on the intended use and the product to be manufactured. This knowledge lies with the manufacturer of the product, not with an authority. A "GMP authority" can therefore not take responsibility for the design of the equipment or confirm it as GMP-compliant by means of a "label" or certificate.
Let us return to the question: What is GMP-compliant equipment design?
In principle, this vague requirement can be broken down to four very obvious requirements for pharmaceutical manufacturing equipment:
1. the equipment must not have a negative impact on product quality
2. the equipment must be easy to clean
3. the equipment must comply with the applicable technical regulations
4. the equipment must be suitable for its purpose.
These 4 points can also be found in different wording in numerous guidelines of GMP-relevant authorities, but are still so unspecific that they require further explanation.
What does "The system must not negatively influence the product quality" mean?
This means that there must be no interaction between the surfaces in contact with the product and the product itself that could negatively affect its quality. Substances may not be released by the system, nor may components of the product be adsorbed. Likewise, no chemical reactions may take place on the surface. The equipment must also not release any substances that could damage the quality of the product; the key words here are lubricants, rust or abrasion. The latter can probably never be 100% avoided, just as material exchange between an internal surface and the product will never be zero. The question here is rather what is tolerable without negatively affecting the product quality. This depends both on the type of material and thus the type of substances that can leak out, and on the type of product.
What does "the equipment must be easy to clean" mean?
There are two objectives here. Firstly, surfaces (in most cases the internal surfaces in contact with the product) should be smooth. Cleaning the equipment by wiping or rinsing is much easier and more effective with smooth surfaces than if the surface has grooves or cracks. For one, bacteria can survive well in these grooves and resist cleaning and even disinfection or sterilization, and product residues can also remain in grooves and gradually pass into subsequent products. This is referred to as cross contamination. The possible survival or remaining of microorganisms in cracks or grooves plays a role above all in the production of sterile pharmaceuticals. The problem here is that microorganisms, unlike chemical contaminants, can multiply. A handful of bacteria has become many millions after half a day, which can mean microbial contamination of the product manufactured in the facility. And even if no viable germs end up in the product at the end of the day, it is their metabolic products or cellular components that can cause major problems. A generally accepted value for surface roughness (the smoothness) is 0.8 μm. Seal sites can also be problematic, i.e., small gaps between the seal and the sealing site.
Even more serious than surfaces that are too rough are places in the system that are difficult to reach. Most systems are cleaned by rinsing or automatically by means of CIP (Cleaning in Place) systems. So-called dead spaces are feared here, i.e. places in the system that are not wetted by the cleaning medium (spray shadows) or to which the cleaning medium cannot reach. In plant engineering, this is referred to as "dead legs". The flow through dead legs is poorer, they are therefore more difficult to clean, and during thermal sanitization it takes longer for these "branches" to also reach the set temperature. Of course, larger quantities of a previous product are more likely to remain in parts of the system that are difficult to clean - keyword cross contamination, see above. This risk is much higher in multipurpose systems than in so-called dedicated equipment, i.e. systems in which only one product is manufactured.
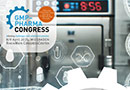
Recommendation
Wiesbaden, Germany8/9 April 2025
GMP PharmaCongress & GMP PharmaTechnica
What does "The equipment must comply with the applicable technical regulations" mean?
Genuine, legally binding GMP regulations are very unspecific, especially when it comes to technical matters. For one thing, the authors are usually not engineers, and for another, as already described, the products manufactured and the equipment used are so varied that generally applicable and at the same time concrete specifications are almost impossible. Furthermore, the GMP specifications would always have to be changed if technological innovations were to occur. The following therefore applies: the equipment must comply with the state of the art, the requirement of, for example, good cleanability still applies, even if new cleaning agents, cleaning processes or similar should become available. So the question is, which technical rules have to be observed or where can one read up on the current state of the art? Of course, this depends on the type of equipment. In a few cases, the pharmacopoeias or guidelines from GMP-relevant authorities with an advisory, i.e. non-mandatory, character are helpful here. It is also always a good idea to know the relevant ISO standards or (in Germany) VDI guidelines. Often, interest groups publish documents that contain further information, e.g. the ECA, the ISPE, the PDA or the EHEDG. The pharmaceutical industry also uses standards from the food industry, especially when it comes to cleanability. It is important to know that these rules are rarely binding. They are nevertheless very often used, as this saves the need to prove that one's own chosen procedure complies with the rules and leads to an equal or better result.
What does "The equipment must be suitable for its purpose" mean?
This is one of the most important and yet least universally understood specifications. It means that the product produced in the equipment meets all pre-determined quality requirements and thus has the effect that the patient needs (and that has been previously proven in a clinical trial). The suitability of the equipment is proven by the qualification of the equipment, which plays an important role in process validation. Again, qualification is product specific. Equipment that is suitable (qualified) for product A does not necessarily have to be equally suitable for product B. Through re-qualification, this suitability must be shown again and again regularly. The pharmaceutical company must prove through qualification that the equipment is suitable for the intended purpose or use. The pharmaceutical manufacturer does not have to carry out the qualification themselves. Often the qualification is sold with the equipment as a package. However, the pharmaceutical company remains responsible for this under pharmaceutical law. An important part of the qualification as well as the recurring measures, which are to maintain the qualified condition of the system, is the calibration. Here, too, the requirements are dictated by the demands of the product. For a very temperature-sensitive product, the temperature measurement must be much more accurate than for a less sensitive product.
These four explanations now shed a little more light on the question of what "GMP-compliant equipment design" is all about. But it should be a little more concrete. This is where a new guidance document has recently come to the rescue, with numerous tips and interpretations of official requirements. The guidance was developed by a task force that included experts in pharmaceutical technology and engineering. The Task Force initially developed a document in German and it was first published by CONCEPT HEIDELBERG. Although the book "GMP Equipment Design Guide" cannot show the requirements for all conceivable manufacturing equipment or replace a technical study, it can be a guide through the thicket of GMP equipment design, create understanding and provide more detailed procedures for the respective individual case or refer to further literature or sources.
The new document is divided into 13 chapters. In chapters 1-4, in addition to an understanding of GMP-compliant system design, the pharmaceutical principles of risk analysis and documented evidence of a GMP-relevant issue are elaborated. Chapters 5 to 13 provide guidance on specific sub-aspects of equipment as they may be used in the manufacture of pharmaceuticals.
1. Introduction: what does GMP-compliant equipment design mean?
2. Overview: general GMP rule works, standards, interest groups and state of the art
3. Risk analysis: the key to pharma success?
4. Evidence: URS, FDS -> GMP design -> qualification
5. Material selection for hygiene critical areas
6. Surface requirements: surface quality grades and surface treatments
7. Hygienic design - basic aspects
8. Piping and fittings; joints, welding & seam control
9. Process environment requirements: the clean room
10. Electrical, instrumentation and control (EI&C) in the GMP-regulated environment
11. Requirements for automation and control systems
12. Documentation in the life cycle of GMP equipment
13. Quality assurance systems required by the equipment supplier
A brief explanation of some of the more detailed chapters follows below.
"Hygiene-critical" in the heading of Chapter 7 refers to areas or products for which there are microbiological requirements. This applies to all pharmaceutical products; for sterile drugs, of course, the hygiene risk is much greater than for solid drugs intended for oral administration.
Two types of materials are widely used in pharmaceutical equipment manufacturing: Polymers and stainless steels. As metallic materials, stainless steels usually exhibit these basic properties. For pharmaceutical applications, stainless steels with the material designation 1.4301/AISI 304, 1.4401/AISI 316, 1.4404/AISI 316L or 1.4435/AISI 316L have become widely established. In principle, metal surfaces in contact with the product may be modified by a surface treatment or coating. However, the surface modification used must also completely fulfill all the basic material properties mentioned above. In other words, no substances may be absorbed or released, no reactions may occur on the surfaces, etc.
Some guidelines contain more detailed information on material selection. These help the user to specify the generally applicable material requirements. One example is the EHEDG (European Hygienic Engineering and Design Group), which has published various guidelines on hygienic equipment design. The most important overarching guide is Document 8. For example, for material selection for stainless steels, this document lists some background information such as the influence of pH, temperature and chloride ion content on possible corrosion. For polymers, the document lists possible polymers such as POM, perfluorinated polymers such as PFA, FEP and ETFE, PVDF, PC, PEEK, PE-HD, PP, PVC, PSU, PPSU and PESU. Common PTFE is critical due to its porosity, as this property can result in insufficient cleanability depending on the application. A selection of elastomers is also mentioned: EPDM, FKM, FFKM, HNBR, NR, NBR and silicone rubber can be used in the food industry.
However, the choice of materials for hygienic production areas is not limited to the equipment. The surrounding area must also be considered. Walls, ceilings and floors can become sources of contamination if the wrong materials are selected. The EHEDH has also published a document on this subject, and Chapter 9 is also devoted to the cleanroom.
Since both the adsorption and desorption of substances and reactions on the surface of the manufacturing equipment may occur, the surfaces in contact with the processed product have a particular influence on its quality. For this reason, surface treatment processes and surface qualities of metallic materials, as they are mostly used in pharmaceutical system engineering, have to be considered in detail.
From a technical-scientific point of view, surfaces can be described by three characteristics: topography, morphology and energy level. In pharmaceutical and biotechnological plant engineering, the consideration of selected togographic and morphological features has become established. In this context, the ASME BPE and the DIN standards for stainless steel components for aseptic applications in the chemical and pharmaceutical industries are particularly worthy of mention. Here, visual inspection with regard to geometric irregularities (defects) and impurities, such as oil and grease residues, corrosion products and water spots, are mentioned, among other things, as are key figures for surface roughness. The surface profilometry method is the most widely used measurement method for determining surface roughness. In pharmaceutical equipment manufacturing, an Ra value of ? 0.8 ?m has become established as a specification. However, there is little scientifically proven data on the significance of the Ra value for the operating behavior of a surface in contact with the product. However, experience from pharmaceutical and biotechnological equipment manufacturing since the early 1990s shows that surfaces with Ra values in the range of 0.1-0.8 ?m do not exhibit any measurable differences with regard to the risk of corrosion, the accumulation of microorganisms and cleanability, provided that the materials and machining processes are the same. Various methods are available for treating the surface: mechanical, chemical and electrochemical processes, with which different surface finishes can be achieved. According to the current state of the art, the minimum requirements are met by surface finishes H3 and H4 to DIN 11866 ff. and SF1, SF2 and SF3 to ASME BPE. These surface grades are suitable for surfaces in contact with processes and products. For higher requirements on corrosion resistance, surface cleanliness and cleanability, the electropolished surface grades HE3 and HE4 to DIN 11866 ff. or SF4, SF5 and SF6 to ASME BPE should be used.
Another important aspect of equipment design is the connection of system parts or systems to each other, i.e. the connection technology, such as the welding of pipes. In addition to the actual connection, the geometry also plays a role here. For example, pipes must be installed with a minimum gradient so that no stagnant liquids can form in the pipe - the keyword here is residual drainability of systems or system components.
An old standard DIN EN729-1 (1994) contains the important statement: "Quality cannot be tested into a product... Even the most comprehensive and sophisticated non-destructive testing does not improve the quality of a weld". Great attention is therefore paid in pharmaceutical plant engineering to welding, or the quality of welds. The most important method is tungsten inert gas (TIG) welding. There is no European guideline or standard that would fully meet the requirements of pharmaceutical & biotech plant engineering. Therefore, the ASME-BPE is usually used. As early as 1997, this standard defined very useful and clear quality criteria for welding work in such a way that they can be used on the construction site.
The quality of a weld seam is judged by the discoloration or tarnish of the weld seam. Since the introduction of automatic welding machines with automatic residual oxygen measurement, defects no longer occur. Further criteria are the through-welding, the uniformity of the seam width and height, the absence of cracks, pores, inclusions or alignment errors.
Automatic orbital welding of stainless steel tubing is state of the art in GMP equipment construction. Special cases are difficult, such as when there is no space for the welding gun and the seam has to be welded by hand. Here, particularly qualified and certified personnel are required - although, unfortunately, there is no "official training certificate" for this to date.
However, even if the quality is to be provided by the welding, the inspection of the weld seams plays a major role. This is done by means of endoscopy, which has become much easier and better in the last 30 years due to the availability of endoscope equipment. The scope of this inspection must be specified, such as 100% of the manual welds and 10-30% of the orbital welds. In addition to the permanent connection, the welding, there are also detachable connections in GMP systems. These are essentially: the dairy coupling, the clamp (or "tri-clamp"), the sterile connection and other manufacturer-specific solutions. The so-called dairy coupling is often found in the food industry. Due to the high viscosity of many products, pipes there often have to be opened for cleaning, which is very easy to do with a hook wrench.
The main disadvantage of the dairy coupling is the gap in the sealing geometry. As a result, this connection is generally no longer used in new pharmaceutical equipment. Only GMP systems which are regularly disassembled for cleaning still use such components in isolated cases. The most commonly used detachable connection in the GMP area is the clamp according to DIN 32676 / ISO 2852. It is also colloquially referred to as Tri-Clamp, but this is a protected brand name of the company Tri-Clover, which sells these components. In sterile technology, connections according to DIN 11864 are used ideally. These are free of dead spaces, but have the disadvantage that the highest processing accuracy is required in pipeline construction.
Let's come back to the less specific requirements in official guidelines when it comes to equipment design. Even if the requirements are brief, unspectacular and, as already mentioned, not very specific, in the GMP environment it is always necessary to prove compliance with requirements or specifications, in other words, to document them. The documentation serves as proof that something has really been done and for the traceability of actions that have been carried out. After all, if errors or deviations have occurred in production, the documentation is used for root cause analysis, to evaluate the effects and to prevent errors in the future. This also applies to the technical documentation of the rooms, plants and equipment, starting with the planning documents and ending with those documents that are required after completion for the smooth operation of a facility.
The qualification documentation is extremely important, as it provides evidence that the facility is suitable for your (GMP) purpose. This starts with the user requirements (URS / User Requirement Specification) and continues via the supplier's design specification (FDS) into the other documents of the various qualification phases IQ, OQ and PQ.
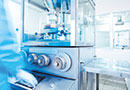
Recommendation
Wiesbaden, Germany8/9 April 2025
Trends in Barrier Systems & Robotics
Important documents that arise in the context of a qualification and are therefore subject to GMP are P&I diagrams, isometrics with weld documentation and testing, lists of devices and machines with process engineering data, lists of measuring points, cable diagrams, wiring diagrams and active circuit diagrams, functional diagrams and floor plans.
The material certificates should also be mentioned here. These ensure that the equipment was built from the materials required by the pharmaceutical manufacturer (as is well known, the material of the facility must not have any negative product influence). If the pharmaceutical manufacturer cannot carry out the material testing or identification himself, this is where the material certificates come into play. For metallic materials, these are the certificates according to DIN EN 102004: Certificate 2.2 (non-specific test) and 3.1 (conformity of the material with the order, stating results of specific test). As far as possible, a 3.1 certificate should be required, as this is the only way to ensure traceability of the certificate to each component. In the case of polymer components, this is often not possible, so that certificates in which the manufacturer himself confirms compliance with the requirements are generally accepted here.
Material certificates for polymers that come into contact with products and media (such as seals) are certificates of conformity issued by manufacturers confirming that they have complied with certain standard or regulatory requirements. In the case of polymers and elastomers, these are in particular requirements originating from the food sector, i.e. guaranteeing suitability for foodstuffs. As a rule, conformity means that the material is not toxic if swallowed. Of course, such a certificate of conformity cannot guarantee general compatibility with the product, process media, etc. Declarations of conformity according to CFR 21 177.2600 Food (US FDA) are well known here. For substances that could unintentionally get into the product (e.g. lubricants), stricter requirements apply. The classification according to USP (US Pharmacopoeia) should be mentioned here, as well as DIN EN ISO 10993. Here, too, conformity is confirmed by the manufacturer (supplier).
About the Author:
Dr Robert Eicher is Operations Director and organises and conducts courses and conferences on behalf of the ECA Academy around pharma technology.